Over the past several years, advances in RNA sequencing have led to an increased appreciation of the prevalence and function of noncoding RNAs, including long noncoding RNAs (lncRNAs). These are typically expressed in a tissue-specific manner in healthy tissues and are often dysregulated in disease, making them potential biomarkers and therapeutic targets. On page 662 of this issue, Li et al. (1) reveal the biological importance of a lncRNA in an inherited metabolic disorder called phenylketonuria (PKU) and demonstrate in mice that a molecule that mimics the functional region of this lncRNA is a promising therapeutic. This discovery suggests that short lncRNA fragments could overcome some of the challenges faced by other RNA therapeutic modalities.
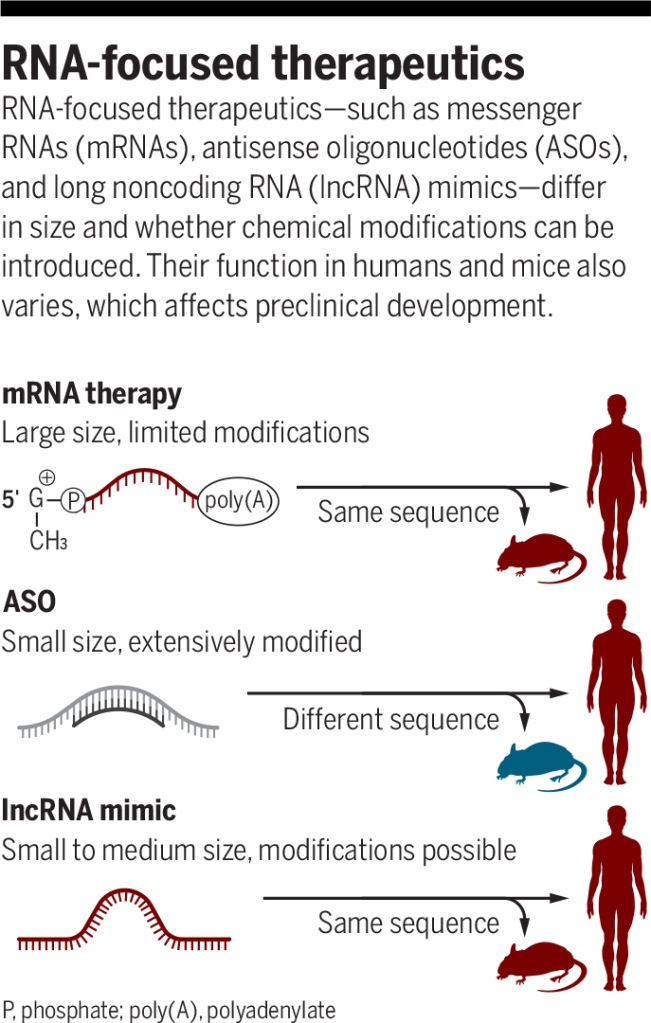
RNA-based and RNA-targeting therapeutics have many advantages: They are cost-effective, are relatively simple to manufacture, can target otherwise undruggable pathways, and have demonstrated success in the treatment of several diseases. Although RNA therapeutics have a long and bumpy history, advances in the generation, purification, and cellular delivery of short oligonucleotides and long RNAs have led to regulatory approval of several RNA-focused therapies, including the much-celebrated messenger RNA (mRNA)–based COVID-19 vaccines.
The human genome encodes a large number of RNA molecules that do not encode functional proteins, including tens of thousands that are classified as lncRNAs (2). lncRNAs and mRNAs are virtually identical at the molecular level, although lncRNA production is typically much more tissue specific. Also, lncRNA genes evolve much faster than protein-coding ones (3). lncRNAs have diverse roles, including in gene regulation and as scaffolds for macromolecular assemblies. Some lncRNAs function in cis—that is, in the vicinity of their site of transcription—whereas others are trans-acting, and their function is not affected by their production site within the genome. Because lncRNAs are expressed in a cell-, tissue-, developmental stage–, or disease-specific manner, their modulation could have substantial, but focal, consequences, which are expected to be well tolerated. However, the progress in elucidating their functions and causally linking genetic changes in lncRNA loci to disease has been slow.
Antisense oligonucleotides (ASOs) are currently the most common approach for therapeutic targeting of RNAs. These are single-stranded oligonucleotides that base pair with a target RNA and can either lead to target degradation or alter target RNA structure and/or its ability to interact with other factors. Chemical modifications of ASOs make them highly stable and able to permeate cells, and considerable progress has been made in the improvement of their pharmacological properties, allowing development of effective therapeutics such as nusinersen for spinal muscular atrophy (4). However, the limited sequence conservation of lncRNAs between human and mouse poses a substantial challenge, because many human lncRNAs do not have recognizable mouse orthologs (3). For those that are conserved, it is often impossible to find an ASO sequence that will recognize both the human and the mouse sequences, which substantially complicates preclinical drug development.
In other cases, increased lncRNA expression is sought, either because the lncRNA is mutated in a disease or because an increase in its concentration carries benefits. One conceptual challenge is that for lncRNAs that function in cis, exogenous delivery to the entire cell will likely not sufficiently increase their concentration at the target locus and may hence remain inconsequential. In any case, a major challenge is the delivery of a large RNA molecule. This can be potentially overcome by identifying and using a functionally active fragment of the full lncRNA. Such a functional element can be a region in the lncRNA molecule that is responsible for interacting with other factors, possibly resulting in changes to their abundance or activity.
For example, the lncRNA Nron (noncoding repressor of NFAT) was identified in mice as a critical suppressor of bone resorption, which is a pathological mechanism in osteoporosis (5). Delivery of full-length Nron using a bone-resorption surface-targeting nucleic acid delivery system inhibits bone resorption but causes side effects in mice, including splenomegaly, probably because of a strong immune response to the delivered RNA. However, the delivery of just the conserved functional motif of Nron, which binds the E3 ubiquitin ligase cullin-4B, effectively reversed bone loss in mice without any obvious side effects, indicating its potential translational use in osteoporosis (5).
Li et al. developed a therapeutic strategy based on the activity of the HULC (hepatocellular carcinoma up-regulated long non-coding RNA) lncRNA which, as they demonstrate, increases the activity of phenylalanine hydroxylase (PAH), which is mutated in PKU. They used lncRNA mimics containing a short fragment of HULC sequence that is tagged with an N-acetylgalactosamine (GalNAc) moiety that facilitates delivery to hepatocytes. Two different lncRNAs, Pair and HULC, perform this function in mouse and human liver, respectively, yet both were able to function equivalently in cells from both species, and the mimics of the functional region in human HULC were effective in vivo at improving PAH function in the mouse liver, without any detectable adverse effects on liver or kidney function.
The use of mimics of lncRNA functional motifs to treat human disease has several advantages compared with other approaches (see the figure). In contrast to therapeutic mRNAs, which need to be translated by ribosomes, and similarly to ASOs, lncRNA mimics can be extensively modified, which can facilitate high in vivo stability and decrease immunogenicity. They can also be easily tagged with organ-targeting peptides for tissue-specific distribution. Functional RNA motifs often do not have strict sequence requirements, which allows flexibility in designing lncRNA mimics and minimizing undesired activities, such as triggering antiviral pathways that recognize different RNA modalities. Because endogenous lncRNA activities are often tissue specific, there is, in principle, a relatively low potential for toxicity. Lastly, as exemplified by Li et al., functional elements can have conserved functions even if their sequences are entirely different, and so the same element can be equivalently active in humans and mice, overcoming a major challenge for ASOs.
Several hurdles still need to be overcome before lncRNAs or fragments thereof realize their full therapeutic potential. Perhaps most important is the need for advances in the methods to deliver RNA molecules to specific tissues and cell types (as nanoparticles or through other vehicles), which will also benefit therapeutic mRNAs and ASOs (6). The repertoire of lncRNAs whose biology is properly understood and linked to specific pathological states also needs to be expanded. Lastly, for as long as the delivery of full-length lncRNAs remains a challenge, new approaches will be needed in computational and/or experimental identification of lncRNA functional domains and of minimal backbones that will facilitate stability and desired subcellular localization.
References and Notes
↵Y. Li et al., Science 373, 662 (2021).
↵M. K. Iyer et al., Nat. Genet. 47, 199 (2015).
↵I. Ulitsky, Nat. Rev. Genet. 17, 601 (2016).
↵X. Shen, D. R. Corey, Nucleic Acids Res. 46, 1584 (2018).
↵F. Jin et al., Nat. Commun. 12, 3319 (2021).
↵M. D. Buschmann et al., Vaccines 9, 65 (2021).