RNA modifications, discovered decades ago, have important biological functions. The most functionally validated modification is the 5′ m7G cap of mRNAs that controls canonical translation (Wei et al., 1975). A wide variety of modifications are present in tRNAs that affect its folding as well as translation. In the past decade, there has been an explosion in the number of known RNA modifications, particularly in mRNAs, and the discovery of their biological roles has spawned the important field of epitranscriptomics (Nachtergaele and He, 2018). In this issue in Cell, Flynn et al., link glycol- and RNA biology with the discovery of a new biopolymer, glycoRNA, a class of RNAs that are glycosylated with sialic acids and fucose (Flynn et al., 2021)
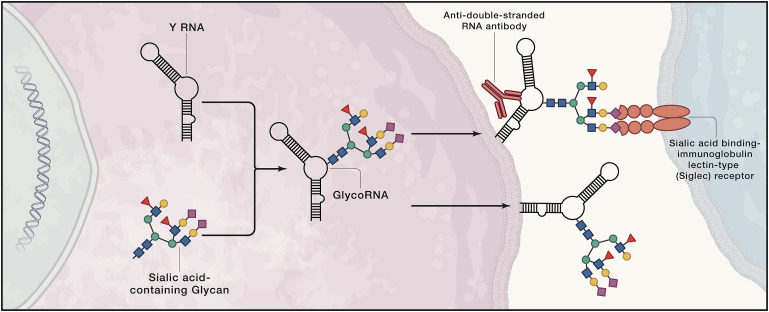
Paradigm shifts often require the development and implementation of tools to dissect and study dark spaces in biology. One approach for isolating, analyzing, and imaging glycosylated biomolecules is to co-opt the cellular biosynthesis of glycans by providing N-azidoacetylmannosamine (Ac4ManNAz), allowing researchers to label sialic-acid-containing glycans with a bioorthogonal handle (Baskin et al., 2007; Saxon et al., 2002). Although used broadly to detect glycosylated proteins, Flynn et al. report the first use of this approach to probe glycosylated RNAs. Indeed, glycoRNAs were detected in various human cell lines and in mouse liver and spleen tissues.
Although many modified mRNAs are found in the epitranscriptome, glycosylated mRNAs were not found. Rather, glycoRNAs are small nuclear (sn)RNAs, ribosomal (r)RNAs, small nucleolar (sno)RNAs, tRNAs, and Y RNAs, the latter of which comprise the greatest percentage of glycosylated RNA species. Further, fractionation and immunohistochemical imaging studies revealed that glycoRNAs are mainly associated with the cell surface, experimentally supported by their loss from the cell surface upon treatment with an enzyme the cleaves sialic acid (Figure 1). That Y RNAs are glycosylated is particularly interesting. Small, conserved RNAs that form ribonucleoprotein complexes, Y RNAs are known antigens associated with autoimmune diseases such as lupus. Because of this disease association and conservation, a series of rigorous experiments were completed to validate Y RNA glycosylation. In particular, CRISPR-Cas9 knockout of Y RNAs in HEK293T cells, which did not affect cell growth as expected from previous studies, ablated Ac4ManNAz-labeling of cells.
Next, the authors investigated whether the same biosynthetic machinery that produces N- and O-linked glycans used to glycosylate proteins also glycosylate RNA. They employed both genetic and pharmacological inhibition approaches. In cells where the glycan biosynthetic machinery is impaired by genetic manipulation, production of glycoRNA is impaired, which can be reversed by supplementation with exogenous glycan. Pharmacological inhibition of oligosaccharyltransferase also diminishes production of glycoRNA. Each study supports that the glycan biosynthetic machinery also produces cellular glycoRNA.
Expression of glycoRNA on the cell surface suggests it may play a role in signaling. It has been assumed that all cell-surface interactions of sialic acid binding-immunoglobulin lectin-type (Siglec) receptor family is due to its binding to glycolipids or glycoproteins. The Siglecs are the largest family of sialoside-binding proteins in humans, and they have important roles in various diseases, from cancers to autoimmune disorders to host-pathogen interactions. Flynn et al. show that two members of the Siglec family (−14 and −11) (Crocker et al., 2007) have interactions with the cell surface that are sensitive to RNase treatment, suggesting that glycoRNA mediate these interactions (Figure 1).
Armed with knowledge of the biopolymer, these rigorous and thorough studies lay the foundation to investigate the exact architecture and structure of glycoRNA; how the glycans are synthesized and incorporated into RNA, which RNAs are subject to glycosylation; and the regulation of its biosynthetic pathway. Most importantly, the precise biological functions of glycoRNAs can be determined. It was only a few decades ago that both RNA and glycans were an afterthought as direct players in human biology. Now that they have chemically joined forces, we should look forward to learning about how glycoRNAs affect biological processes!
References
J.M. Baskin, J.A. Prescher, S.T. Laughlin, N.J. Agard, P.V. Chang, I.A. Miller, A. Lo, J.A. Codelli, C.R. Bertozzi Copper-free click chemistry for dynamic in vivo imaging. Proc. Natl. Acad. Sci. USA, 104 (2007), pp. 16793-16797
P.R. Crocker, J.C. Paulson, A. Varki Siglecs and their roles in the immune system. Nat. Rev. Immunol., 7 (2007), pp. 255-266
R.A. Flynn, K. Pedram, S.A. Malaker, P.J. Batista, B.A.H. Smith, A.G. Johnson, B.M. George, K. Majzoub, P.W. Villalta, J.E. Carette, et al. Small RNAs are modified with N-glycans and displayed on the surface of living cells. Cell, 184 (2021), pp. 3109-3124
S. Nachtergaele, C. He Chemical modifications in the life of an mRNA transcript. Annu. Rev. Genet., 52 (2018), pp. 349-372
E. Saxon, S.J. Luchansky, H.C. Hang, C. Yu, S.C. Lee, C.R. Bertozzi. Investigating cellular metabolism of synthetic azidosugars with the Staudinger ligation J. Am. Chem. Soc., 124 (2002), pp. 14893-14902
C.M. Wei, A. Gershowitz, B. Moss. Methylated nucleotides block 5¢ terminus of HeLa cell messenger RNA Cell, 4 (1975), pp. 379-386